Undoubtedly, space exploration has occupied an important place within the interests of humanity within in the last 50 years. Names like Challenger, Apollo or Columbia are not alien to any person with access to radio or television who has lived during the decades between the 70s and 90s. All these names are part of the achievements of the humanity in the 20th century during the heyday of space exploration.
Both manned and cargo trips have been possible thanks to a long chain technological and scientific advances that have nurtured space agencies since the beginnings of the space race until its later years. Engineers and scientists from all over the world have put their efforts in improving both the reliability and safety of the mission, such as optimising equipment to make these trips at a lower cost possible, leading them to explore the most innovative technologies and interesting, including solid fuel rocket propulsion.
Solid fuel rockets are used like space shuttle accelerators during the first two minutes of flight. These will mainly case of a housing, a insulation layer, nozzle, load propellant (solid fuel) and a lighter to start the ignition of the system. The biggest novelty of this propulsion system lies precisely in the type of fuel that is used (in solid state), which gives many advantages versus propulsion systems with liquid fuels.
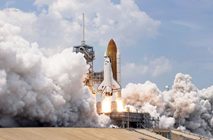
One of the most important points is the high density of these fuels compared with other liquid fuels, being able to store a greater amount of "energy" within the same geometry. The fact that the fuel is in state solid and noticeably less volatile than liquid fuels, it makes its storage is safer and easier, being its handling much simpler and reducing both construction and disposal costs.
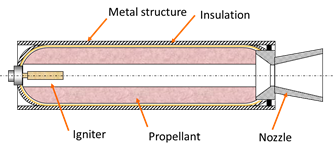
Figure 2. Solid rocket booster sketch
The "propellant" material is a mixture of a oxidizing agent, a material to be oxidized and a binder. Ammonium perchlorate is generally used (NH4ClO4) as an oxidizing agent, aluminium atomized as fuel (Al) and HTPB (polybutadiene with hydroxyl radicals) as a binder (CxHyOz). The reaction between these compounds generates different gases, which within the combustion can reach up to 3400 [K] temperature and 2200 [K] when expelled by the nozzle (given the expansion that occurs).
The nozzle design is what allows the exit of gases, which in turn also regulates the burning rate of solid fuel, encapsulated in the principal structure. These can escape through the nozzle at speeds up to 1500 m / s, generating the thrust needed to accelerate, for example, a shuttle space in conjunction with the two rockets of acceleration that commonly constitute the typical launch setup. This set weighs approximately 2000 tons.
Depending on the cross section of the fuel distribution in the rocket is how it will carry the momentum during acceleration. Since combustion occurs in the rocket centre area and the momentum depends directly on the rocket's burning area, the geometry of the central "bore" determines the type of flight the thruster can give. For example, if the geometry consists of a circle (tubular, see figure 3), the impulse type It will be progressive, since the combustion area will increase as the fuel is burns, so the impulse will increase with time, while in a multifin (n°4 in figure 3) initially the burning area is larger, but as the burning radius increases, the area does not increase greatly, maintaining the constant momentum for most of the flight.
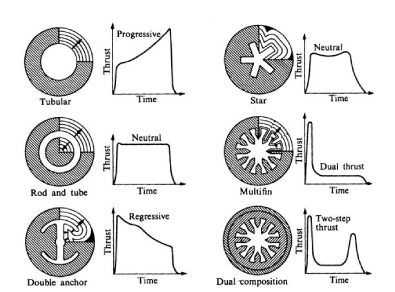
Figure 3. Internal combustion load designs with their impulse time programs.
The oxidation of solid fuels is highly energetic, for example, if it is taken to a comparative scale in fuels, the oxidation of Aluminum (Al) gives 83,781 MJ / m3, while propane generates 99 MJ / m3, generating 846 times les energy per cubic meter of fuel. In figure 4 you can see the proportional differences of solid fuels (Al and Fe) with those of gaseous fuels (hydrogen, methane and propane).
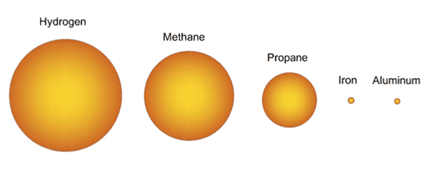
Figure 4. Proportion of volume of fuel required for a given amount of energy.
In addition to the characteristic of high calorific value per unit volume of solid fuels it is important to know that reactions occur in different states (solid, liquid or gaseous) depending on the material, for example, the oxidation of aluminium is in the liquid state (> 2000 ° C) and that of iron is in solid state (870 ° C). This allows define applications of different types, as is the case of thermal lances that are used to cut or tap punch any existing material.
Using the energy of a solid that reacts in this state, as is the case of iron, has a very relevant application in foundry processes, since to pierce the plugs that hold the molten material (liquid) within the furnace require materials with high resistance to heat energy. To pierce these plugs, the industry has been developing different technologies for more than 130 years without achieving until today a product that can effectively open 100% of these plugs, different to thermal lances.
From all this it could be said that spaceships take advantage of the transformation of the high thermal energy of solid fuels into high kinetic energy resulting in a large thrust, while in the case of foundries the high solid state thermal energy of iron is used to concentrate it in a precise point and melt the plug. In both situations, the expected result would not be achieved with conventional fuels.
Curiously, the optimisation of thermal lances goes through developments of configurations very similar to the space rocket nozzles, as shown in Figure 5.
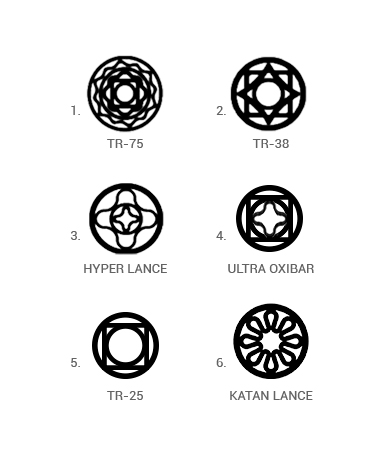
Figure 5. Profiles of some Trefimet thermal lances.