Among all the branches of physics that have become popular in recent times, quantum mechanics undoubtedly occupies a special place among them. In this area, nature is studied on the scales of the order of atomic systems, subatomic particles and their interactions with each other.
The visible universe consists of about 100 different elements. However, every day it is possible to find countless chemical compounds in such an everyday space as an office worker's desk. This is because it is naturally very difficult to find atoms that stand alone. They are usually found in various combinations with atoms of other elements or in pairs of the same element by means of chemical bonds. The obvious question is: Why isn't the universe full of just these 100 different atoms, instead of the infinite number of chemical compounds that inhabit it?
The answer lies in understanding the role of energy in the formation of molecules and its basis in quantum mechanics.
Many of the most important substances for us, such as air, for example, consist not of individual atoms but of compounds. Oxygen is not found in nature individually, but as two atoms bonded together (O2), as is nitrogen (N2) or more complex compounds such as the water molecule (H2O). The systems just named, like the rest, tend to adopt the minimum energy state. To understand this better. We will take it to the following example:
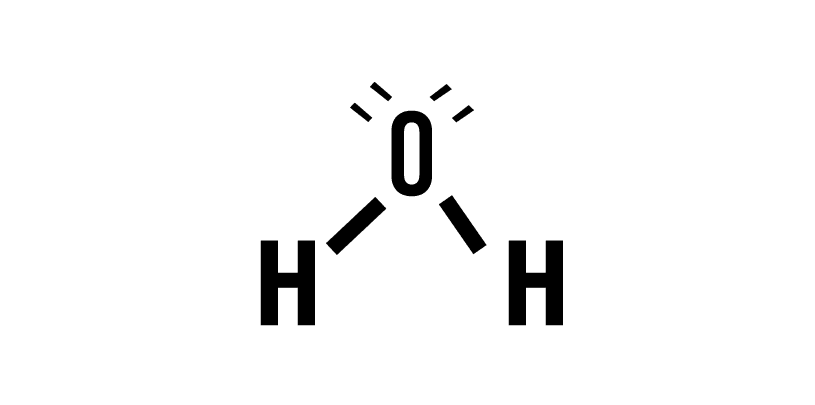
The hydrogen atom by itself will be in its lowest energy state, called the ground state. But when a second hydrogen atom is introduced, interesting phenomena begin to happen. Both atoms are in their respective ground states, but as they approach each other, their electrons and protons begin to generate attractions and repulsions due to their respective electric charges. All these interactions have an associated energy to take into account when calculating the minimum energy state, which are determined by the Schrödinger equation.
Although the Schrödinger equation defines the minimum energy states, it is not trivial to solve and many mathematical tools must be applied to generate conclusions from it. One very important fact that can be seen from the solutions to the equation is that the energy state of a system of two atoms is lower than the energy of two separate systems of one atom. This is why, if two hydrogen atoms are close to each other, they will naturally combine to form a molecule ofH2.
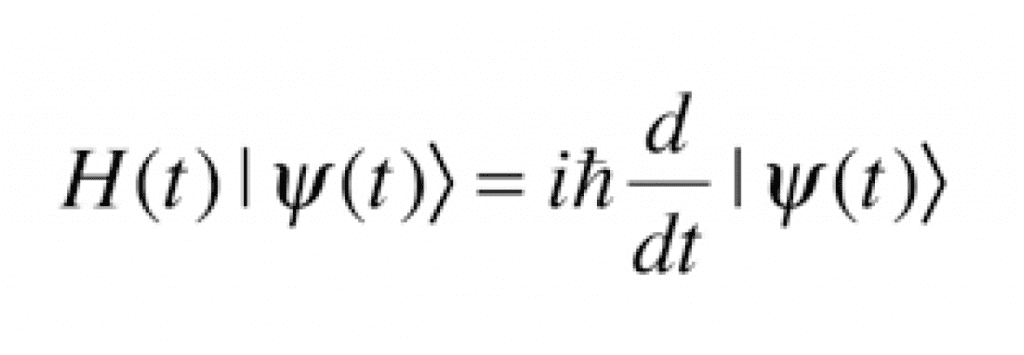
But not all elements form bonds with atoms of their own kind, or with any other atom. All substances strive for stability by sharing or having a specific number of electrons: 2, 10, 18, 36, 54 or 86 in the orbitals around the nucleus. These numbers correspond to the number of electrons contained in the 6 noble gases.
In particular, iron tends to share the extra electrons in its outermost shell in an oxidation process. This gives rise to several types of iron oxides where each has a different level of stability from the other and is closer to the lowest energy state. The state of the resulting compound has so little energy compared to the initial states that a large amount of energy is released into the environment as a result of this reaction. This is commonly called an exothermic reaction. The energy levels are such that one cubic metre of iron can release 57 447 000 kilo Joules, if it is oxidised to its lowest energy state (Fe2O3), enough energy to power the engine of a 25 tonne truck over a journey of 4268 kilometres (across the length of Chile).
While in the case above it is said that the oxidation states of iron could be used to utilise the energy released (mechanical energy), there are more grounded cases where this exothermic energy is used, and this is the case of thermal lances.
By coexisting diatomic oxygen with low carbon steel, the oxidation of the latter is promoted. Although the oxidation process of steel in general is a non-spontaneous process, by injecting energy into the system, the valence electrons (electrons in the last shell) become excited and are more likely to react, achieving the lower energy state more violently, thus making the reaction spontaneous.
The energy released in the thermal lances is used to melt different materials to be pierced or cut, and as a result of the reaction, iron oxides and heat energy are obtained. Different oxides can be obtained since, like all processes or events described in quantum mechanics, these are probabilistic, so that, although a large majority of the iron tends to go to the lowest energy state (Fe2O3), other iron particles will pass through an intermediate oxidation state before the final one, or will not even reach the state of minimum energy (degrees of least oxidation).
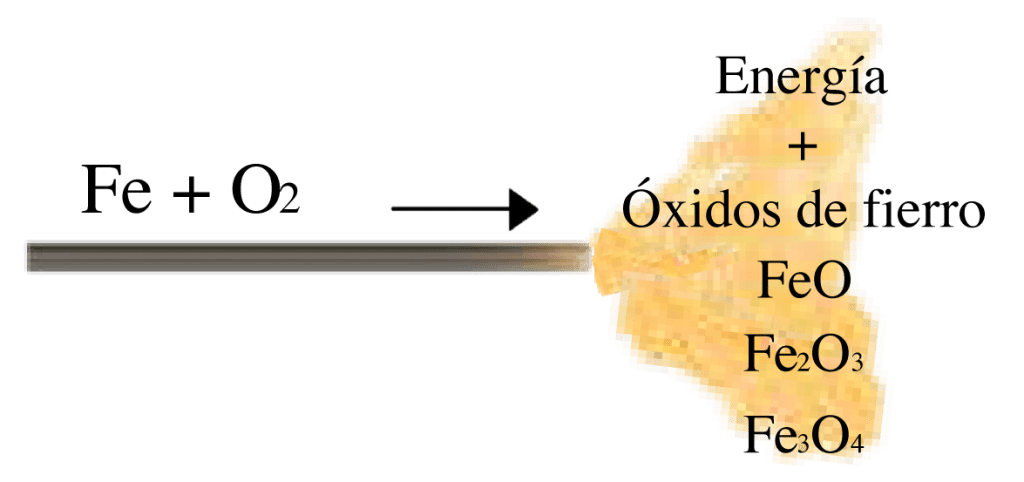
Although quantum mechanics, which is behind all these phenomena, allows us to see how nature behaves, it does not explain why nature is the way it is. Why things work the way they do is one of the most interesting questions to answer, and one to which many scientists have devoted much of their lives.
Richard Feynmann (Nobel Prize in physics in 1965) once said "I think I can safely say that nobody understands quantum mechanics".a statement that to this day has not been disproved. However, this has not been a reason to put aside this wonderful branch of physics, which to this day has made it possible to determine phenomena that have made many engineering developments possible, such as the theorisation of all liquid fuels and solid fuels, including thermal lances.